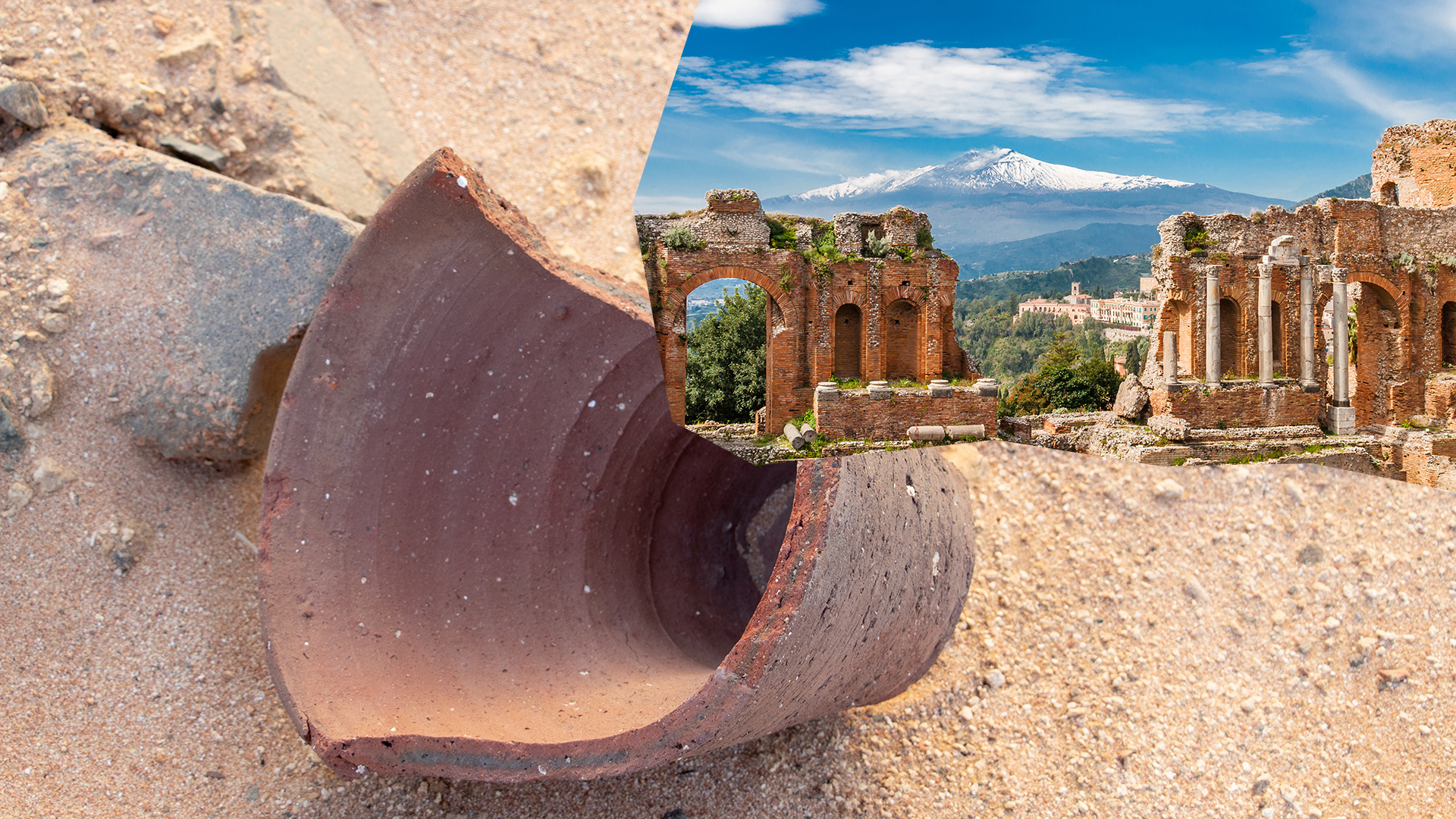
Discovering how our ancestors used to live
Using HPLC-MS and GC-MS approaches in archaeology to reveal ancient lipid species
Discovering how our ancestors used to live
Using HPLC-MS and GC-MS approaches in archaeology to reveal ancient lipid species
Valentina Chiaia, PhD Danilo Donnarumma, PhD Giuseppe Micalizzi, PhD Anna Irto,
Prof. Clemente Bretti, Prof. Paola Cardiano, Prof. Luigi Mondello, University of Messina
Lipids are the main chemical class of biomolecules studied in ancient pottery, due to their stability over time compared to other organic compounds such as carbohydrates and proteins. However, alteration processes such as thermal decomposition, oxidation and hydrolysis may occur. An artificial ageing approach has been performed to simulate the degradation processes of lipids occurring in archaeological pottery. Lipid species were separated and identified by using HPLC-MS and GC-MS methodologies.
Reviving ancient daily life by tracing lipids in pottery
The analyses of organic residues in archaeological pottery are fundamental to unveiling crucial hints about the daily life of ancient societies. Pottery vessels absorb organic material and preserve it totally or partially during burial over millennia, hereby providing valuable insight about the use of various natural resources. Analytical investigations for traces of organic matter, visible organic residues, surface deposits or encrustations can establish not only their origin but also the function and the use of the pottery vessels. This allows addressing a wide range of questions on ancient human activities.
The attention of analysts is particularly focused on lipid compounds due to their greater stability over time compared to other organic compounds such as carbohydrates and proteins [1]. The hydrophobic character of lipids, in fact, limits their percolation over the centuries and lets them stay in the original site. On the other hand, more polar substances are more susceptible to decay, especially those containing nitrogen and phosphorous atoms [2]. Although the hydrophobic character of lipids could make them excellent candidates for “archaeological biomarkers” [3], the presence of reactive functional groups along the molecular structures may lead to their alteration. In addition, the preservation of lipid matter strongly depends on the environmental condition (e.g., dry climate preserves lipids to a greater extent [4]) and on the material in which they are trapped. Highly dense or vitrified materials reduce the microbial degradation due to their low porosity and permeability, while clay surfaces containing high quantity of water and other reactive species may cause some chemical degradation processes, such as hydrolysis or oxidation [5].
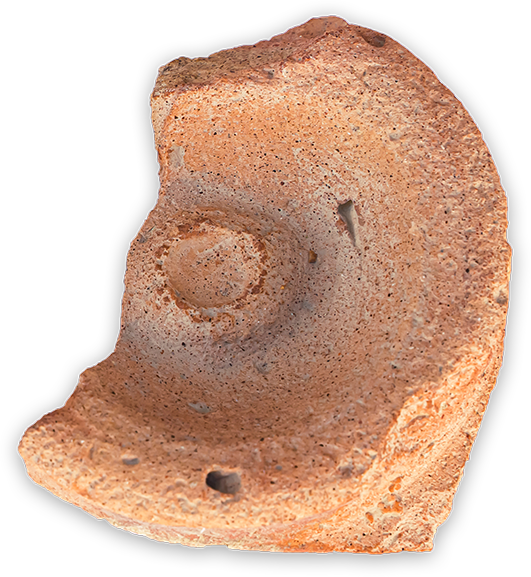
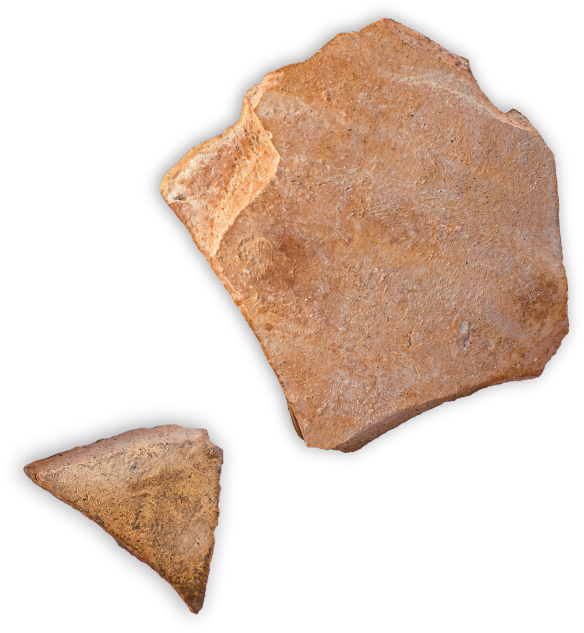
A peek in the pots of ancient cuisine – distinguishing marine and terrestrial products
The simulation of thermal decomposition, oxidation or hydrolysis of lipids can provide key elements to better interpret the origin of animal fats and vegetable oils partially or totally altered over time [3]. In the past, accelerated ageing studies have already been carried out in order to simulate lipids’ natural alteration occurring in the archaeological site. For instance, Colombini et al [6] established that main oxidation products of monounsaturated fatty acids (MUFAs) as gondoic acid (C20:1n9) and erucic acid (C22:1n9), particularly abundant in Brassica juncea seed oil, were (α,ω)-undecanedioic and (α,ω) tridecanedioic acids. This means that the molecular structure of oxidation products strongly depends on the location of the double bond along the carbon chain of the native fatty acid. Moreover, Bondetti et al. [7] proposed an innovative criterion to distinguish marine and terrestrial natural products based on the molecular structure of long‐chain ω‐(o‐alkylphenyl)alkanoic acids (APAAs), which are oxidation products of fatty acids containing multiple double bonds. For example, APAA with 20 and 22 carbon atoms could be directly related to the cooking of aquatic organisms [8], since they derive from eicosapentaenoic acid (C20:5n3) and docosahexaenoic acid (C22:6n3), which are particularly abundant in aquatic sources.
Combining GC and HPLC with MS for the separation of aged lipid species
Ageing studies generally involve the use of chromatographic techniques with a high resolving power coupled to mass spectrometry (MS). The alteration/degradation products are initially separated based on their interaction with mobile and stationary phases and then detected in the MS dimension. The relative retention time (linear retention index, LRI) and fragmentation pattern can therefore be used in order to unambiguously reveal the identity of unknown lipid compounds. The main aim of this research study is the development of analytical methodologies as gas chromatography (GC) and high-performance liquid chromatography (HPLC) coupled to mass spectrometry (MS) for the separation and identification of aged lipid species. In-lab thermal-oxidative treatments have been carried out in order to simulate the natural degradation of olive oil, traditionally used in Mediterranean ancient societies.
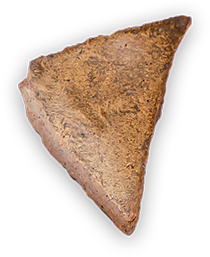
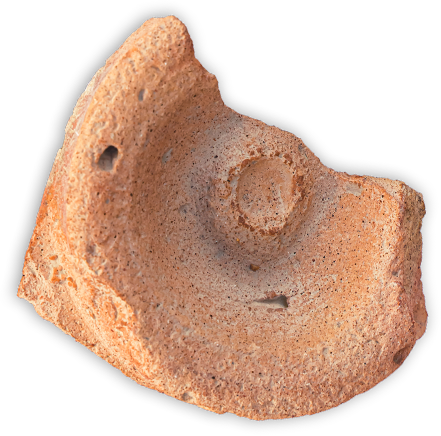
Materials and methods
Tristearin (SSS), triolein (OOO) and trilinolein (LLL) standards (10 mg) were kept in an oven at 120 °C for three weeks. The thermo-oxidative treatment was also performed
on 10 mg of extra-virgin olive oil.
Aged intact lipids were initially diluted in 2-propanol (1,000 mg L-1) and 1 μL was directly injected into an Ultra High-Performance Liquid Chromatograph (UHPLC) Nexera X2 system, including two LC-30 AD dual-plunger parallel-flow pumps, a DGU-20A5R degasser, a CTO-20AC column oven and a SIL-30AC autosampler.
The UHPLC system was coupled to a LCMS-8060 triple quadrupole mass spectrometer equipped with APCI interface. The separation was achieved by Non-Aqueous Reversed Phase High-Performance Liquid Chromatography (NARP-HPLC) using 2 Ascentis Express C18 columns (100 mm L × 2.1 mm ID, 2.7 μm particle size). The mobile phase consisted of: (A) acetonitrile and (B) 2-propanol, with a flow rate of 0.4 mL min-1.
Chromatographic separation was performed according to the following gradient elution: 0–105 min, 0–50% B, held 20 min. MS parameters were as follows: m/z range 250–1200; event time 0.2 s; nebulizing gas (N2) flow rate 3 L min-1; drying gas (N2) flow rate 5 L min-1; interface temperature 350 °C; DL temperature 200 °C; heat block temperature 200 °C.
Data were collected and processed using the LabSolution software. For LRI calculation, the odd carbon number triacylglycerol (TG) mixture from C9C9C9 to C17C17C17 was used as reference homologue series [9].
GC analyses
Aged lipids were initially converted into trimethylsilyl (TMS) derivatives in order to increase volatility and reduce polarity. In this respect, all samples were derivatized with 200 μL of N,O-bis(trimethylsilyl)trifluoroacetamide (BSTFA) reagent containing 1% of trimethylchlorosilane (TMCS) and 500 μL of dichloromethane (CH2Cl2). Then, the reaction mixture was heated for 20 min at 80 °C. Separation and identification of fatty acids were performed on a GCMS-QP2020 NX system.
The injection of the samples was carried out in an automatic way by using an AOC-20i autosampler. A split/splitless injector was installed on the GCMS instrument. The separation of TMS derivatives was achieved by using a non-polar capillary column, namely SLB-5ms (equivalent in polarity to poly-5% diphenyl/95% dimethyl siloxane-phase) 30 m x 0.25 mm ID x 0.25 μm df. The temperature program was: 50 °C to 360 °C at 3 °C min-1. Injection volume and split ratio were: 0.5 μL in splitless mode. Helium was used as carrier gas with a constant linear velocity of 30 cm sec-1. MS parameters were: electronic ionization (70 eV); ion source temperature: 250 °C; mass range: m/z 40–650; interface temperature: 250 °C. A C7–C40 saturated alkanes standard mixture was used for LRI calculation. The GCMSsolution software was used for both data acquisition and processing.
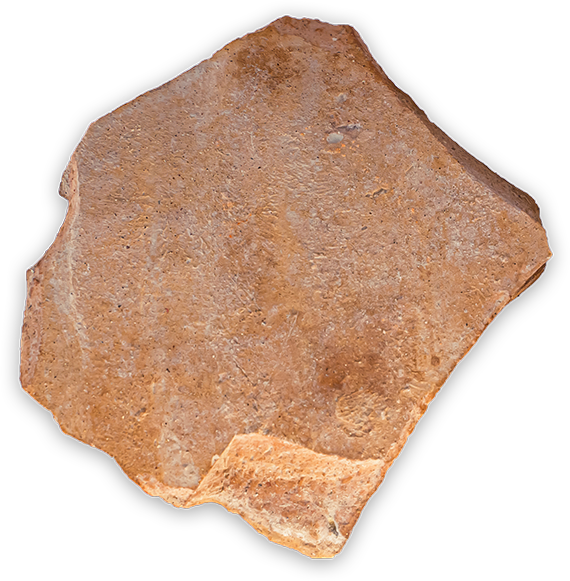
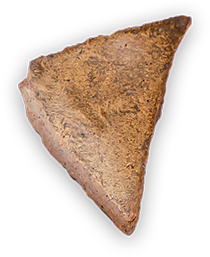
Results and discussion
In the present work, a NARP-HPLC method coupled to MS via APCI interface was applied for the analysis of intact lipids in TG standards and extra-virgin olive oil subjected to thermal-oxidative treatments. Figure 1 shows the HPLC-MS chromatograms of the intact lipids in not aged and aged olive oils. Elution of the lipid species occurs in accordance with the increase in the partition number (PN), derived from the equation PN = CN – 2DB, where CN and DB are the number of carbon atoms and the number of double bonds of fatty acid, respectively. Lipid species were identified according to Oteri et al. [9], in which two different identification criteria, MS fragment patterns and LRIs, were used. In Table 1 are listed the identified lipids and retention times (RT), detected ions, experimental and reference LRIs for each species. A lab-constructed database containing LRI values was utilized to evaluate the correspondence of experimental LRIs.
NARP-HPLC-APCI-MS analysis revealed the formation of oxidized lipid species, characterized by a mass increasing of 16 Dalton (molecular weight of an oxygen atom) as reported in Table 1.
RT |
[M-H2O+H]+ |
[M+H]+ |
[M+NH4]+ |
Diagnostic fragments |
Class |
CN:DB |
Compound |
PN |
LRIexp |
LRIref |
Not aged |
Aged |
7.92 |
593.5 |
611.5 |
313.3 355.3 |
Ox. DG |
34:1 |
Ox. PO |
32 |
2,844 |
– |
nd |
x |
|
8.28 |
619.5 |
637.5 |
339.3 355.3 |
Ox. DG |
36:2 |
Ox. OO |
32 |
2,865 |
– |
nd |
x |
|
12.13 |
575.5 |
313.3 337.3 |
DG |
34:2 |
PL |
30 |
3,089 |
– |
x |
nd |
||
12.13 |
601.5 |
337.3 339.3 |
DG |
36:3 |
OL |
30 |
3,089 |
– |
x |
nd |
||
16.72 |
577.5 |
313.3 339.3 |
DG |
34:1 |
PO |
32 |
3,327 |
– |
x |
x |
||
16.72 |
603.5 |
339.3 |
DG |
36:2 |
OO |
32 |
3,327 |
– |
x |
x |
||
47.84 |
899.7 |
916.7 |
617.5 619.5 |
Ox. TG |
54:4 |
Ox. OOL |
46 |
4,144 |
– |
nd |
x |
|
49.31 |
873.7 |
890.7 |
591.5 593.5 617.5 |
Ox. TG |
52:3 |
Ox. POL |
46 |
4,178 |
– |
nd |
x |
|
49.68 |
879.7 |
896.7 |
597.5 599.5 601.5 |
TG |
54:6 |
OLLn |
42 |
4,187 |
4,192 |
x |
nd |
|
52.38 |
875.7 |
593.5 619.5 |
Ox. TG |
52:2 |
Ox. POO |
48 |
4,249 |
– |
nd |
x |
||
53.07 |
901.7 |
918.7 |
619.5 |
Ox. TG |
54:3 |
Ox. OOO |
48 |
4,265 |
– |
nd |
x |
|
54.05 |
849.7 |
593.5 |
Ox. TG |
50:1 |
Ox. PPO |
48 |
4,288 |
– |
nd |
x |
||
56.65 |
881.7 |
898.7 |
599.5 601.5 |
TG |
54:5 |
OLL |
44 |
4,348 |
4,342 |
x |
nd |
|
57.44 |
855.7 |
872.7 |
575.5 599.5 |
TG |
52:4 |
PLL |
44 |
4,366 |
4,358 |
x |
x |
|
58.25 |
855.7 |
872.7 |
573.5 577.5 599.5 |
TG |
52:4 |
POLn |
44 |
4,385 |
4,383 |
x |
nd |
|
64.28 |
883.7 |
900.7 |
601.5 603.5 |
TG |
54:4 |
OOL |
46 |
4,528 |
4,516 |
x |
x |
|
65.16 |
857.7 |
874.7 |
575.5 577.5 601.5 |
TG |
52:3 |
POL |
46 |
4,551 |
4,539 |
x |
x |
|
66.06 |
831.7 |
848.7 |
551.4 575.4 |
TG |
50:2 |
PPL |
46 |
4,574 |
4,571 |
x |
nd |
|
71.69 |
885.7 |
902.7 |
603.5 |
TG |
54:3 |
OOO |
48 |
4,722 |
4,729 |
x |
x |
|
72.73 |
859.7 |
876.7 |
603.5 577.5 |
TG |
52:2 |
POO |
48 |
4,749 |
4,756 |
x |
x |
|
73.81 |
833.7 |
850.7 |
551.5 577.5 |
TG |
50:1 |
PPO |
48 |
4,777 |
4,776 |
x |
x |
|
78.91 |
913.8 |
930.8 |
603.5 631.5 |
TG |
56:3 |
OOG |
50 |
4,911 |
4,905 |
x |
nd |
|
80.23 |
887.7 |
904.7 |
603.5 605.5 |
TG |
54:2 |
SOO |
50 |
4,945 |
4,948 |
x |
x |
Table 1: List of lipid species identified in not aged and aged olive oils (x: detected; nd: not detected)
Note: Ox. DG: oxidated diacylglycerol; DG: diacylglycerol; Ox. TG: oxidated triacyl glycerol; TG: triacylglycerol.
Abbreviations: RT: retention time; [M-H2O+H]+, [M+H]+, [M+NH4]+ and diagnostic fragments: detected ions; Class: lipid class; CN : DB: carbon number: double bond; PN: partition number; LRIexp: experimental LRI; LRIref: reference LRI.
Figure 1: HPLC-MS chromatograms of intact lipid detected in not aged (upper) and aged (bottom) extra-virgin olive oils
Note: Ox. DG: oxidated diacylglycerol; DG: diacylglycerol; Ox. TG: oxidated triacylglycerol; TG: triacylglycerol
Abbreviations: P: palmitic acid; S: stearic acid; O: oleic acid; L: linoleic acid; Ln: linolenic acid; A: arachidic acid; G: gondoic acid
Figure 1: HPLC-MS chromatograms of intact lipid detected in not aged (upper) and aged (bottom) extra-virgin olive oils
Note: Ox. DG: oxidated diacylglycerol; DG: diacylglycerol; Ox. TG: oxidated triacylglycerol; TG: triacylglycerol
Abbreviations: P: palmitic acid; S: stearic acid; O: oleic acid; L: linoleic acid; Ln: linolenic acid; A: arachidic acid; G: gondoic acid
A significant loss of the most abundant TGs, such as triolein (OOO), was observed, probably related to the formation of free fatty acids as confirmed by the GC analyses.
GC-MS analyses confirmed that thermo-oxidative treatments caused the formation of high levels of short-chain fatty acids (SCFAs) containing a number of carbon atoms ranging from 5 to 9 units. Hexanoic (C6:0), heptanoic (C7:0), octanoic (C8:0) and nonanoic (C9:0) acids were the most abundant SCFAs in aged olive oil as shown in the GC-MS chromatograms illustrated in Figure 2. The thermo-oxidative treatment implied the inclusion of an oxygen atom in the carbon chain, the scission of the double bond and formation of lower molecular weight species, as also reported by Colombini et al. [6]. For instance, the detection of C9:0 in aged olive oil was due to the scission of the double bond in the C9-position of the oleic acid (C18:1n9), while the high amount of C6:0 was due to the scission of the double bond in the C6-position along the carbon chain of the linoleic acid (C18:2n6) (Figure 2).
With regards to tristearin, the GC-MS chromatogram (not reported) revealed the high stability of the compound due to absence of double bonds along the carbon chain. Therefore, fatty acids containing at least one double bond are more susceptible to the oxidative stress. Finally, (α,ω)- dicarboxylic acids such as adipic, pimelic, suberic, azelaic and sebacic acids were also detected in aged samples (Figure 2), according to literature data [6]. Considering their lower volatility compared to SCFAs, (α,ω)-dicarboxylic acids may be considered as potential archaeological biomarkers, although further investigations will be needed to find characteristic biomarkers for olive oil. In Table 2 the list of fatty acids (as TMS derivatives) detected in analyzed samples is reported.
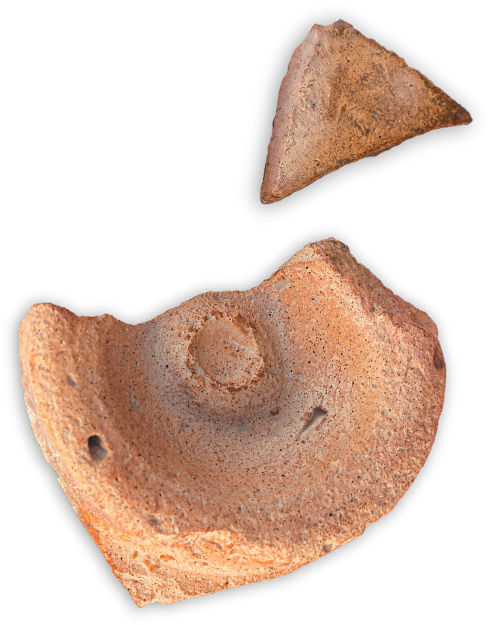
Compound |
MS similarity |
LRIexp |
LRIref |
Pentanoic acid; TMS. C5:0 |
89 |
980 |
974 |
Hexanoic acid; TMS. C6:0 |
93 |
1,072 |
1,069 |
Heptanoic acid; TMS. C7:0 |
93 |
1,167 |
1,166 |
Octanoic acid; TMS. C8:0 |
92 |
1,262 |
1,262 |
Succinic acid; 2-TMS. C4:0 |
92 |
1,312 |
1,310 |
2-Octenoic acid; TMS. C8:1n6 |
93 |
1,316 |
– |
Nonanoic acid; TMS. C9:0 |
89 |
1,359 |
1,358 |
Pentanedioic acid; 2-TMS. C5:0 |
90 |
1,404 |
1,403 |
Decanoic acid; TMS. C10:0 |
90 |
1,456 |
1,456 |
Adipic acid; 2-TMS. C6:0 |
88 |
1,504 |
1,503 |
Pimelic acid; 2-TMS. C7:0 |
90 |
1,602 |
1,601 |
Suberic acid; 2-TMS. C8:0 |
88 |
1,696 |
1,694 |
Azelaic acid; 2-TMS. C9:0 |
91 |
1,795 |
1,792 |
Sebacic acid; 2-TMS. C10:0 |
90 |
1,891 |
1,889 |
Palmitelaidic acid; TMS. C16:1n7 |
96 |
2,023 |
– |
Palmitic acid; TMS. C16:0 |
89 |
2,045 |
2,043 |
Linoleic acid; TMS. C18:2n6 |
96 |
2,207 |
– |
Stearic acid; C18:0 |
93 |
2,168 |
– |
Oleic acid; TMS. C18:1n9 |
93 |
2,215 |
2,207 |
Cis-vaccenic acid; TMS. C18:1n7 |
95 |
2,222 |
2,226 |
Stearic acid; TMS. C18:0 |
97 |
2,243 |
2,237 |
Arachidic acid; TMS. C20:0 |
96 |
2,440 |
2,437 |
Table 2. List of fatty acid compounds detected as TMS derivatives in aged samples
Abbreviations: MS similarity: mass spectral similarity; LRIexp: experimental LRI; LRIref: reference LRI
Uncovering the secrets of ancient life left behind
Both HPLC-MS and GC-MS analytical methodologies were used for the elucidation of the main oxidation and degradation products in extra-virgin olive oil subject to the thermo-oxidative treatment. Two different identification criteria, MS fragment patterns and LRI, were used to reveal the exact identity of chromatographic peaks. Such strategy will allow to determine putative archaeological biomarkers, useful to better interpret the origin of animal fats and vegetable oils used by ancient societies that are partially or totally altered over time. In particular, the HPLC-MS approach allowed to evaluate the oxidation at intact lipids level, while the GC-MS methodology was pivotal for the identification of species at low molecular weight such as SCFAs and (α,ω)-dicarboxylic acids. The applied approaches resulted to be particularly valid and reliable for the characterization of altered/degraded lipid species in archaeological context.
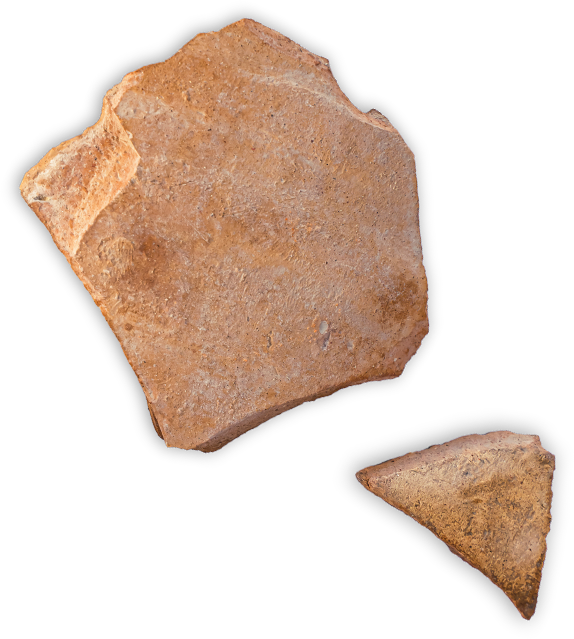
Literature
- Stern, B.; Heron, C.; Tellefsen, T.; Serpico, M., New investigations into the Uluburun resin cargo. J. Archaeol. Sci. 2008, 35, (8), 2188-2203.
- Evershed, R.P. Organic residue in archaeology: The archaeological biomarker revolution. Archaeometry. 2008, 50, 895–924.
- Irto, A.; Micalizzi, G.; Bretti, C.; Chiaia, V.; Mondello, L.; Cardiano, P. Lipids in Archaeological Pottery: A Review on Their Sampling and Extraction Techniques. Molecules. 2022, 27, 3451.
- Copley, M.S.; Bland, H.A.; Rose, P.; Horton, M.; Evershed, R.P. Gas chromatographic, mass spectrometric and stable carbon isotopic investigations of organic residues of plant oils and animal fats employed as illuminants in archaeological lamps from Egypt. Analyst. 2005, 130, 860–871.
- Jones, M.K.; Briggs, D.E.G.; Eglington, G.; Hagelberg, E.; Evershed, R.P.; Dudd, S.N.; Charters, S.; Mottram, H.; Stott, A.W.; Raven, A.; et al. Lipids as carriers of anthropogenic signals from prehistory. Philos. Trans. R. Soc. Lond. B Biol. Sci. 1999, 354, 19–31.
- Colombini, M. P.; Giachi, G.; Modugno, F.; Ribechini, E. Characterisation of organic residues in pottery vessels of the Roman age from Antinoe (Egypt). Microchemical Journal. 2005, 79, 83–90.
- Bondetti, M.; Scott, E.; Courel, B.; Lucquin, A.; Shoda, S.; Lundy, J.; Labra-Odde, C.; Drieu, L.; Craig, O.E. Investigating the formation and diagnostic value of ω-(o-alkylphenyl)alkanoic acids in ancient pottery. Archaeometry. 2020, 63, 594–608.
- Cramp, L.J.E.; Evershed, R.P. Reconstructing Aquatic Resource Exploitation in Human Prehistory Using Lipid Biomarkers and Stable Isotopes; Elsevier: Oxford, UK, 2014.
- Oteri, M.; Rigano, F.; Micalizzi, G.; Casale, M.; Malegori, C.; Dugo, P.; Mondello, L. Comparison of lipid profile of Italian Extra Virgin Olive Oils by using rapid chromatographic approaches. J. Food Compos. Anal. 2022, 110, 104531.