Closing the loop
on emissions
TOC analysis in the quest to achieve carbon neutrality
Markus Janssen, Shimadzu Europa GmbH
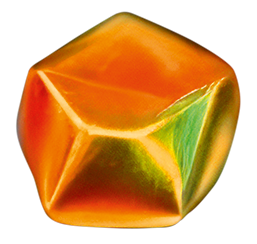
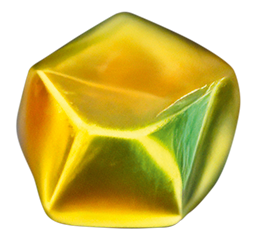
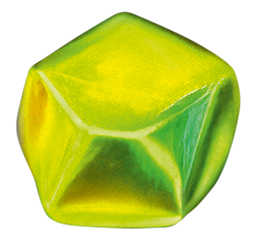
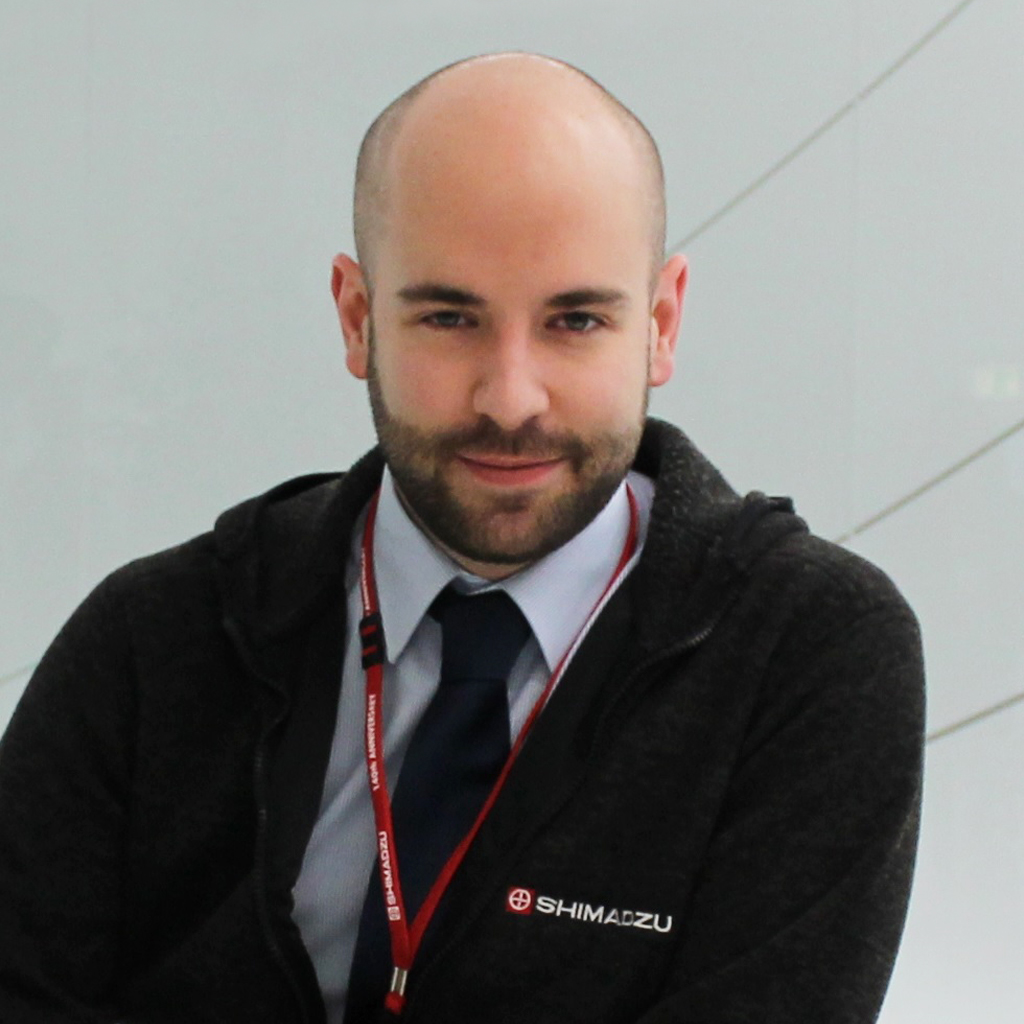
Carbon neutrality, a key goal in the fight against climate change, requires a delicate balance between carbon emissions and removals. Disruptions to the natural carbon cycle intensify climate challenges. Cleaner technologies and innovative Negative Emission Technologies (NETs) are critical to achieving carbon neutrality. TOC analysis plays an important role in quantifying carbon dynamics, evaluating carbon-sequestering materials such as concrete and advancing sustainability. By using TOC analysis to “close the loop on emissions”, effective carbon management becomes a pathway to a greener future.
In the quest to combat climate change and promote a sustainable future, the concept of carbon neutrality has gained significant prominence. Carbon neutrality, often synonymous with net-zero emissions, refers to the state in which the release of carbon dioxide (CO2) and other greenhouse gases into the atmosphere is balanced or offset by the removal or reduction of an equivalent amount. Achieving carbon neutrality is an important and evolving goal, characterized by ongoing research and continuous technological advances. It represents a significant step toward stabilizing the earth’s climate and preserving our planet for future generations.
The pressing issue is that the excessive release of carbon dioxide (CO2) into the atmosphere is largely man-made and is disrupting the natural carbon cycle with alarming consequences. The carbon cycle, a delicate natural process that regulates the flow of carbon between the atmosphere, oceans, land and living organisms, is being disrupted on an unprecedented scale. In a world where this balance is out of control, the consequences of anthropogenic emissions exacerbate the challenges of climate change.
Two complementary ways to achieve carbon neutrality
First, emissions must be reduced at the source through the adoption of cleaner technologies and sustainable practices. The second critical aspect could be the implementation of negative emission technologies (NETs). These innovative approaches focus on removing carbon dioxide from the atmosphere and actively work to offset any remaining emissions. A remarkable feature of NETs is that they mimic natural dynamics in their carbon sequestration mechanisms.
Understanding carbon complexity is key
In order to pursue carbon neutrality, carbon in its various forms must be studied. CO2 is an essential natural component and is not inherently problematic. The oceans, for example, act as vast carbon sinks, absorbing approximately 25 % of CO2 emissions and contributing to dissolved inorganic carbon (DIC), total inorganic carbon (TIC) and total organic carbon (TOC). When CO2 dissolves in water, it forms carbonic acid (H2CO3), which further dissociates into bicarbonate (HCO3–) and carbonate (CO32–) ions. This equilibrium depends on the pH of the water (Figure 1).
TIC includes these inorganic forms of carbon which together represent the sum of carbon for potential gaseous CO2 release under certain conditions. Some carbon remains in the oceans as marine organisms use it to build shells. Over time, these remains can become sedimentary rocks, effectively storing carbon for millions of years. Algae and phytoplankton convert CO2 into biomass, a form of total organic carbon (TOC). As they are consumed by other organisms, organic carbon moves up the food chain, increasing TOC levels in the broader ecosphere. Both TIC and TOC cycles facilitate forms of carbon sequestration.
The NET worth: taking climate change head-on with these methods
Negative emission technologies (NETs) are man-made carbon sequestration methods designed to remove CO2 from the atmosphere or from emission sources. To develop effective NETs, measurement of both total organic carbon (TOC) and total inorganic carbon (TIC) is critical. TOC analyzers, such as the TOC-L and TOC-4200 systems, play an important role in this process because they operate on the principle of the inner carbon cycle. High temperature oxidation converts organic carbon compounds to carbon dioxide (CO2), which can then be quantified using non-dispersive infrared (NDIR) detectors. Next, the pH of the sample is changed to release dissolved carbon dioxide (CO2) from inorganic carbon sources, which can also be quantified by NDIR. This integrated approach provides valuable insights into carbon composition, contributing to the advancement of effective NETs and sustainable carbon management strategies.
Microorganisms as a solution for a large-scale problem
Just like in the book “The War of the Worlds” by H.G. Wells, bacteria might hold the key to preserve our planet and our species. Though it is widely acknowledged that plants and microalgae can use CO2 in photosynthesis to produce their own nutrients, the primitive life form of bacteria could hold distinct advantages over these organisms. They exhibit a significantly accelerated growth rate and life cycle, thrive in densely populated cultures and are readily amenable to genetic manipulation. Moreover, like other microorganisms, bacteria show remarkable potential in producing a diverse range of bio-alcohols and fatty acids essential for various industrial applications, including oil production. By capitalizing on the unique features of photosynthetic bacteria, science and industry alike are exploring innovative approaches for addressing industrial demands and sustainable biotechnological advancements.
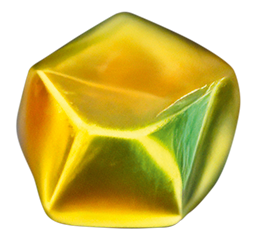
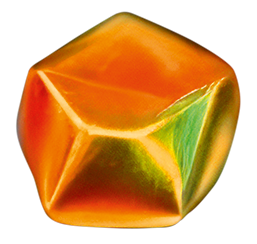
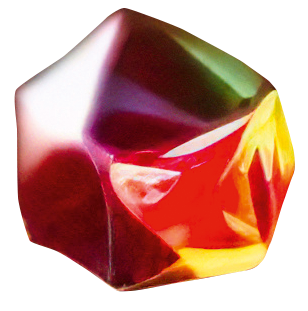
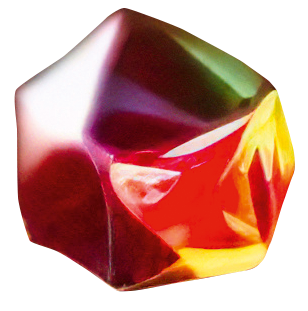
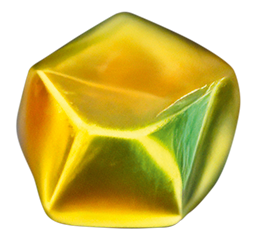
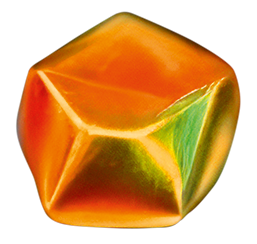
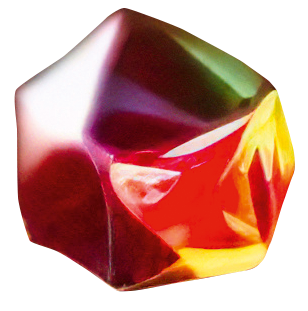
In the controlled environment of a laboratory, the use of a TOC-L analyzer makes it possible to evaluate the uptake of carbon dioxide by microbial metabolism. The initial amount of CO2 introduced into the closed bacterial culture medium was precisely measured by TIC analysis. The experiment was then meticulously replicated after 3 and 24 hours, allowing accurate quantification of the carbon used to increase microbial biomass (Table 1). This detailed approach provides valuable insights into the dynamics of microbial carbon utilization.
Notably, this natural process showcases the inherent ability of microorganisms to actively capture and utilize carbon dioxide. Within a span of 24 hours, an amount of approximately 5 g CO2 per liter of medium could be effectively bound, all the while producing valuable biomass that could be processed into biofuel or other valuable feedstock. The biomass produced can be accurately measured as well using TOC analysis. This allows researchers to precisely quantify the organic carbon content, providing a comprehensive understanding of the microbial biomass generated during the process.
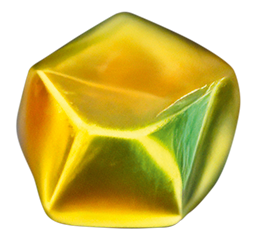
Building materials against climate change: carbon-sequestering concrete
Cement production is a significant source of CO2 emissions (approximately 4.5 % of global emissions [1]), contributing to the greenhouse effect and global warming. CO2 is released during the cement manufacturing process when limestone, a key component of cement, is heated to high temperatures in a kiln to producing clinker. Subsequently, when concrete is produced by mixing cement, water and aggregates, additional CO2 is released as the cement undergoes hydration and hardens.
Carbon-sequestering concrete is a specialized form of concrete that actively captures and stores CO2 during its curing process. This innovative approach involves incorporating materials that react with CO2, such as certain mineral additives, into the concrete mix. As the concrete cures, these materials react with atmospheric CO2 and convert it into a mineral form, effectively storing the carbon within the concrete structure.
TIC analysis is used to evaluate the carbon sequestration capabilities of this concrete. While other methods such as thermal analysis or titration of a hydrochloric acid solution can also be used, they require significant time and effort and can only measure small sample quantities, leading to biased results due to uneven sample distribution. Using the TOC-L with the solid sample measurement system SSM-5000A, milled and dried concrete samples can be analyzed quickly, easily and accurately by simply weighing them into a sample boat for analysis.
In an experiment (Table 2), two types of concrete samples were examined: ordinary concrete and CO2-sequestering concrete. The samples were ground into powder, and 50 mg of each were weighed into sample boats. Phosphoric acid was dripped onto the samples, and the inorganic carbon (IC) content was measured in the SSM-5000A solid sampling module. The CO2-sequestering concrete showed about up to five times higher CO2 absorbing potential than the ordinary concrete, highlighting its effective CO2 absorption capability.
DACCS: Direct Air Carbon Capture and Storage
While efforts to reduce emissions by binding CO2 in products are commendable, they alone may not achieve negative emissions. Direct air carbon capture and sequestration (DACCS) technology, which is still in development, may offer an innovative way to actively remove carbon from the atmosphere and safely store it as stable carbonate rock. The integration of an online total organic carbon (TOC) analyzer provides valuable information on various process steps. It continuously monitors the TOC and TIC content of the DAC fluid before injection into an underground storage site, ensuring that any organic or inorganic impurities are accounted for. This facilitates accurate carbon accounting and evaluation of the overall carbon sequestration process.
The process begins with direct air capture (DAC) technology, which uses specialized equipment to extract CO2 directly from the air. After purification to meet storage standards, the compressed gas is transported to the designated site. There, it is injected into underground rock formations using pumped-up groundwater or seawater. The CO2 is dissolved in the carbonated water, recombines with minerals and over months forms pockets of carbonate rock within the porous basalt that are stable for geologic timespans. Subsurface injection with water eliminates the risk of gas leakage and provides safe long-term carbon storage. Other methods under development involve injecting gaseous CO2 or supercritical fluid into suitable storage sites, such as saline aquifers or depleted oil and gas reservoirs.
With the ability to quantify both organic and inorganic carbon sources, TOC analyzers facilitate the advancement of effective negative emission technologies (NETs) that mimic natural carbon sequestration mechanisms. They provide invaluable insight into carbon dynamics and play a key role in achieving carbon neutrality by evaluating the efficiency of carbon-sequestering materials like concrete. Analyzers with TIC analysis capability, like the TOC-L and TOC-4200, are important in the development and implementation of carbon sequestration and emerging technologies like direct air carbon capture and sequestration (DACCS). By enabling complete accounting of the flow of carbon through TOC/TIC analysis, these analyzers contribute significantly to the creation of a sustainable future. They provide a comprehensive understanding and quantification of carbon dynamics in these processes, thus paving the way for effective carbon management and a greener planet.
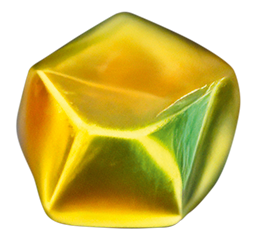
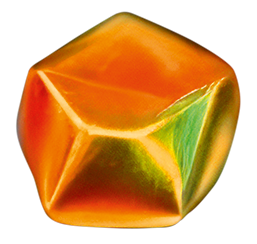
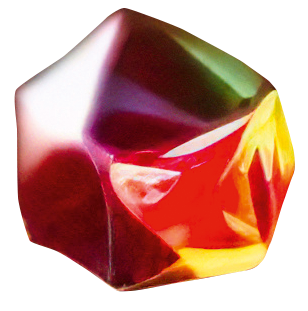
Time passed |
TIC conc. [mg C/L] |
Coefficient of variation [%] |
Total CO2 bound by microbiology [mg/L] |
0 hours |
1,694 |
0.45 |
0.00 |
3 hours |
1,163 |
0.95 |
1,943.46 |
24 hours |
288.3 |
1.26 |
5,144.86 |
[CO2/C]: 44.01 g/mol / 12.01 g/mol = 3.66 |
Sample |
TIC result [wt-% C] |
Coefficient of variation [%] |
CO2 Potential [wt-%] |
Ordinary concrete |
1.41 |
2.96 |
5.16 |
CO2-sequestering concrete |
6.76 |
2.01 |
24.74 |
[CO2/C]: 44.01 g/mol / 12.01 g/mol = 3.66 |
The realignment of humanity with nature
The climate is like a big puzzle messed up by human influence. Recently, the UN has changed the term “global warming” to “global boiling”, redefining the urgency we confront. But this challenge also sparks human creativity, driving us to find answers. By implementing NETs that mimic natural mechanisms, we achieve complete accountability of carbon in sequestration processes (CO2 gas to liquid/solid or CO2 to biomass). This transformation allows us not only to realign our products and our way of life but also ourselves with nature, our essential ally, and natural NET.
[1] Statista: Carbon dioxide emissions from the manufacture of cement worldwide from 1960 to 2021 https://www.statista.com/statistics/1299532/carbon-dioxide-emissions-worldwide-cement-manufacturing/; Annual carbon dioxide (CO₂) emissions worldwide from 1940 to 2022 https://www.statista.com/statistics/276629/global-co2-emissions/